Turbulence, mixing, and shelf sea transports
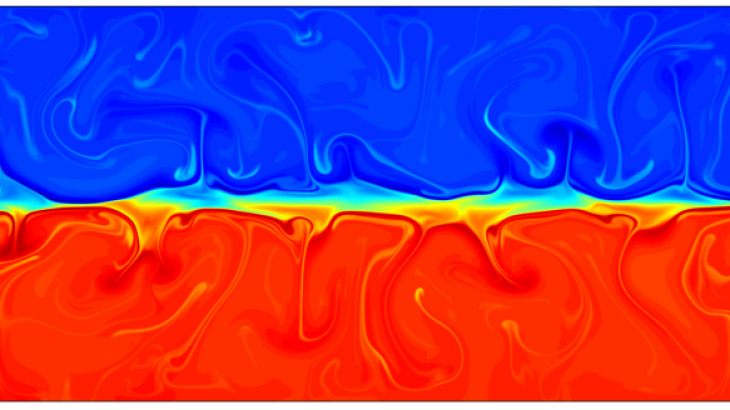
Image of a clear stratification. -image: Hereon-
Although ocean turbulence is a very small-scale motion in the ocean on the order of 1 cm, it is a crucial component of large-scale budgets of energy, momentum, and important scalars such as heat and nutrients. It is the process responsible for driving ocean mixing, which is a key ingredient in setting rates of vertical transport in the oceans.
The often strong vertical stratification of the oceans is a hindrance to vertical motions and transports, which must be accomplished through small-scale turbulent mixing. Mixing exerts control on many processes that are of paramount importance to coastal and shelf seas; from enabling the supply of nutrients to the sunlit surface layer, to the supply of oxygenated waters to the deep Baltic Sea, and the oceanic heat fluxes delivered to the Arctic sea ice.

A high performance computer cluster used for simulations. -image: Hereon-
Recent advances in understanding turbulent mixing and transport in coastal seas have been possible through novel measurement and simulation techniques. These advancements in observational technology have been made in parallel with advances in computational resources, allowing for a strong interplay between in situ field observations and numerical simulations. The use of high performance computer clusters to simulate ocean turbulence with DNS (Direct Numerical Simulations) have led to an advancement in heat transport laws in double diffusive convection, with a link to an improved understanding of the energy budget. Although normally limited to unrealistically low-energy turbulent events that are not present in the oceans, these simulations have crossed into the realm of geophysical turbulence - simulating conditions present in the oceans.
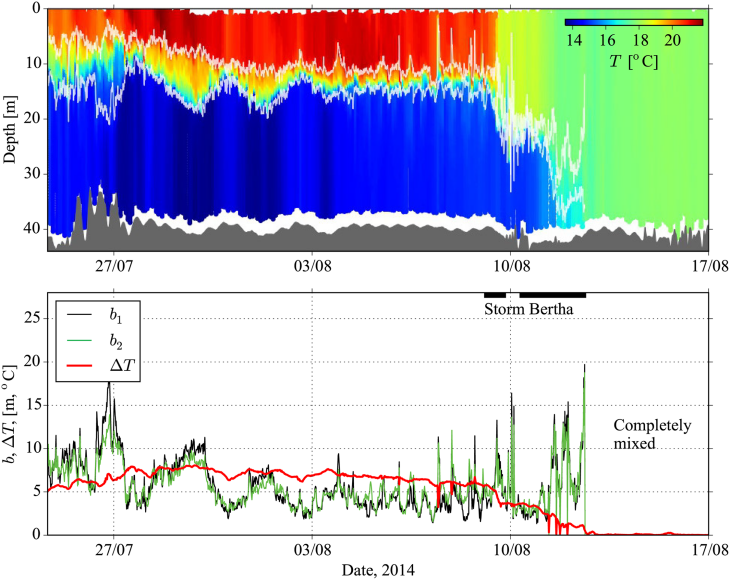
Glider measurements of North Sea stratification, summer 2014. The upper panel shows the conservative temperature in depth and time. The pycnocline region is indicated by the light coloured lines. The bottom panel shows thermocline thickness using two different methods (b1, b2), and total temperature difference, ΔT. The approximate times of Storm Bertha are indicated by the dark bar at the top of the panel. -image: Carpenter JR, Merckelbach L, Callies U, Clark S, Gaslikova L, Baschek B (2016) Potential Impacts of Offshore Wind Farms on North Sea Stratification. PLoS ONE 11(8): e0160830. https://doi.org/10.1371/journal.pone.0160830. CC BY 4.0-
This has enabled a direct, and successful, comparison with in situ turbulence measurements. These results have been used to understand and constrain estimates of Arctic Ocean heat fluxes due to double-diffusive convection. In addition, an investigation is currently underway to identify and quantify these processes in the Baltic Sea, where the ocean gliders were instrumental in discovering double diffusive staircases for the first time.
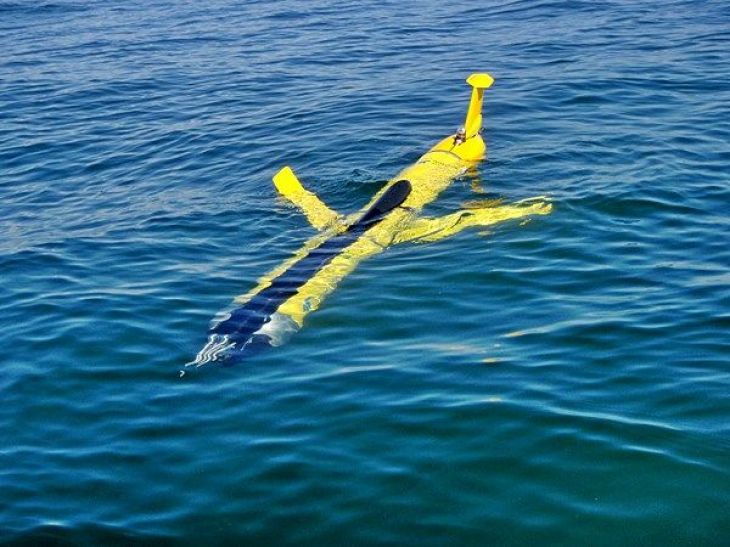
An underwater glider equipped with turbulence sensors. -image: Hereon/Raimo Kopetzky-
This combined approach using both simulation and observation is currently being applied to understand the influences of offshore wind farm (OWF) development on North Sea stratification. Preliminary estimates of the turbulent mixing of OWFs suggest that it could provide a significant impact on stratification, with the large-scale construction of OWFs in the North Sea.
In addition, the glider-based measurements of North Sea turbulence levels suggest that OWFs could contribute to significantly enhanced turbulence in the stratified thermocline region. Given these findings, in situ measurements using towed instrument chains, and Large Eddy Simulations of the stratified turbulent wake of OWF foundation structures are currently underway.
[i] Hieronymus, M. & J.R. Carpenter: Energy and variance budgets of a diffusive staircase with implications for heat flux scaling. Journal of Physical Oceanography, 46, 2553-2569, 2016, doi:10.1175/JPO-D-15-0155.1.
Umlauf, L., Holtermann, P., Gillner, C., Prien, R., Merckelbach, L. & Carpenter, J.R. (2018): Diffusive convection under rapidly varying conditions. Journal of Physical Oceanography, 48, 1731-1747, doi: 10.1175/JPO-D-18-0018.1
Sommer, T., Carpenter, J.R., Wuest, A.: Double-diffusive interfaces in Lake Kivu reproduced by direct numerical simulations,Geophysical Research Letters, 41, 2014 doi:10.1002/2014GL060716.
Shibley, N., Timmermans, M.-L., Carpenter, J.R., Toole, J.: Spatial variability of the Arctic Ocean's doublediffusive staircase. Journal of Geophysical Research - Oceans, 122, 2017, doi:10.1002/2016JC012419.
Flöter, J., van Beusekom, J., Auch, D., Callies, U., Carpenter, J.R., et al.: Pelagic effects of offshore wind farm foundations in the stratified North Sea. Progress in Oceanography, 156, 154-173, 2017, doi:10.1016/j.pocean.2017.07.003.
Carpenter, J.R., Merckelbach, L., Callies, U., Clark, S., Gaslikova L., Baschek, B.: Potential impacts of offshore wind farms on North Sea stratification. PLoS ONE, 11(8), e0160830, 2016,
doi:10.1371/journal.pone.0160830.
Schultze, L. K. P., Merckelbach, L. M. and Carpenter, J. R., Turbulence and Mixing in a Shallow Shelf Sea From Underwater Gliders. Journal of Geophysical Research – Oceans, 122, doi: 10.1002/2017JC012872.